Brutally Hard Math Is Its Own Reward - Russian mathematician Grigory Perelman turned down $1 million for proving the Poincaré conjecture. What's that?
The New York Times recently reported that reclusive Russian geometer Grigory Perelman has apparently proved the century-old Poincaré conjecture. The Times calls Poincaré "a landmark not just of mathematics, but of human thought." But just why it's so significant is left a bit hazy. Big mathematical advances often generate the same kind of lofty but content-free rhetoric found in political speeches about "the family." Like the family, math is a subject everyone agrees is very important without being able to specify exactly why.
I'm here to help. (With the Poincaré conjecture. As for the family, you're on your own.)
Poincaré conjectured that three-dimensional shapes that share certain easy-to-check properties with spheres actually are spheres. What are these properties? My fellow geometer Christina Sormani describes the setup as follows:
The Poincaré Conjecture says, Hey, you've got this alien blob that can ooze its way out of the hold of any lasso you tie around it? Then that blob is just an out-of-shape ball. [Grigory] Perelman and [Columbia University's Richard] Hamilton proved this fact by heating the blob up, making it sing, stretching it like hot mozzarella, and chopping it into a million pieces. In short, the alien ain't no bagel you can swing around with a string through his hole.
That's zingier than anything the Times will run, but may still leave you without a clear picture of Perelman's theorem. Indeed, it's pretty hard to give an elementary account of the statement that Poincaré conjectured and that Perelman seems to have confirmed. (If that's what you're after, Sormani's home page links to a variety of expositions, including one in the form of a short story.) Instead, I'll try to explain why Perelman's theorem matters without explaining what it is.
The entities we study in science fall into two categories: those which can be classified in a way a human can understand, and those which are unclassifiably wild. Numbers are in the first class—you would agree that although you cannot list all the whole numbers, you have a good sense of what numbers are out there. Platonic solids are another good example. There are just five: the tetrahedron, the cube, the octahedron, the dodecahedron, and the icosahedron. End of story—you know them all. These mathematical objects behave something like the chemical elements, which are neatly classified by Mendeleev's periodic table. Many properties of an element are determined by its place in the table. For instance, we knew a lot about how metals like germanium and gallium would behave before they were actually discovered in nature!
In the second class are things like networks (in mathematical lingo, graphs) and beetles. There doesn't appear to be any nice, orderly structure on the set of all beetles, and we've got no way to predict what kinds of novel species will turn up. All we can do is observe some features that most beetles seem to share, most of the time. But there's no periodic table of beetles, and there probably couldn't be.
Mathematicians are much happier when a mathematical subject turns out to be of the first, more structured, type. We are much sadder when a subject turns out to be a variegated mass of beetles. (But have a look at Fields Medalist Timothy Gowers' beautiful essay "The Two Cultures of Mathematics" for a spirited defense of mathematical enterprises of the second sort.)
So, where do three-dimensional shapes, the subject of the Poincaré conjecture, fit in? To simplify, let's think about two-dimensional shapes first. These fall firmly in the "periodic table" category. The only such shapes are the surfaces of "doughnuts" with multiple holes. The number of holes is called the genus of the surface and plays the role that the atomic number does for chemical elements. (Here is a picture of the surfaces of genus 0, 1, 2, and 3.) Geometer William Thurston (another Fields winner) made the daring conjecture that three-dimensional shapes, too, can be classified in a more complicated but equally structured way. Perelman has proved this conjecture, which has Poincaré as a straightforward corollary. That means, in turn, that we can think about proving general statements about three-dimensional geometry in a way that we can't hope to about beetles or graphs.
Perelman's work isn't important because of its applications. It won't help anyone build a bridge, aim a rocket, crack a code, or privatize Social Security. Mathematicians, no dummies, like to point out that, in some unspecified future, Perelman's theorem might pitch in to help with these problems in ways that aren't obvious now. But its real significance is like that of the fact that a times b is equal to b times a; it's a basic structural statement about how the world is organized. If you prefer order to chaos, that's something worth caring about.] ( slate.com )
Russian mathematician Grigory Perelman has turned down a $1 million prize for proving the century-old Poincaré conjecture, one of the world's most difficult math problems. Although Perelman solved the problem in 2003 and was awarded the prize in March, he did not refuse the honor until Thursday, citing disagreements with the "organized mathematical community." In 2006, mathematician Jordan Ellenberg explained the Poincaré conjecture and why it's important. The original article is reprinted below.
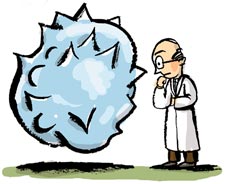
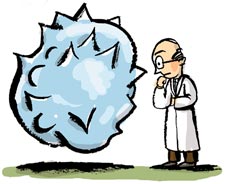
The New York Times recently reported that reclusive Russian geometer Grigory Perelman has apparently proved the century-old Poincaré conjecture. The Times calls Poincaré "a landmark not just of mathematics, but of human thought." But just why it's so significant is left a bit hazy. Big mathematical advances often generate the same kind of lofty but content-free rhetoric found in political speeches about "the family." Like the family, math is a subject everyone agrees is very important without being able to specify exactly why.
I'm here to help. (With the Poincaré conjecture. As for the family, you're on your own.)
Poincaré conjectured that three-dimensional shapes that share certain easy-to-check properties with spheres actually are spheres. What are these properties? My fellow geometer Christina Sormani describes the setup as follows:
The Poincaré Conjecture says, Hey, you've got this alien blob that can ooze its way out of the hold of any lasso you tie around it? Then that blob is just an out-of-shape ball. [Grigory] Perelman and [Columbia University's Richard] Hamilton proved this fact by heating the blob up, making it sing, stretching it like hot mozzarella, and chopping it into a million pieces. In short, the alien ain't no bagel you can swing around with a string through his hole.
That's zingier than anything the Times will run, but may still leave you without a clear picture of Perelman's theorem. Indeed, it's pretty hard to give an elementary account of the statement that Poincaré conjectured and that Perelman seems to have confirmed. (If that's what you're after, Sormani's home page links to a variety of expositions, including one in the form of a short story.) Instead, I'll try to explain why Perelman's theorem matters without explaining what it is.
The entities we study in science fall into two categories: those which can be classified in a way a human can understand, and those which are unclassifiably wild. Numbers are in the first class—you would agree that although you cannot list all the whole numbers, you have a good sense of what numbers are out there. Platonic solids are another good example. There are just five: the tetrahedron, the cube, the octahedron, the dodecahedron, and the icosahedron. End of story—you know them all. These mathematical objects behave something like the chemical elements, which are neatly classified by Mendeleev's periodic table. Many properties of an element are determined by its place in the table. For instance, we knew a lot about how metals like germanium and gallium would behave before they were actually discovered in nature!
In the second class are things like networks (in mathematical lingo, graphs) and beetles. There doesn't appear to be any nice, orderly structure on the set of all beetles, and we've got no way to predict what kinds of novel species will turn up. All we can do is observe some features that most beetles seem to share, most of the time. But there's no periodic table of beetles, and there probably couldn't be.
Mathematicians are much happier when a mathematical subject turns out to be of the first, more structured, type. We are much sadder when a subject turns out to be a variegated mass of beetles. (But have a look at Fields Medalist Timothy Gowers' beautiful essay "The Two Cultures of Mathematics" for a spirited defense of mathematical enterprises of the second sort.)
So, where do three-dimensional shapes, the subject of the Poincaré conjecture, fit in? To simplify, let's think about two-dimensional shapes first. These fall firmly in the "periodic table" category. The only such shapes are the surfaces of "doughnuts" with multiple holes. The number of holes is called the genus of the surface and plays the role that the atomic number does for chemical elements. (Here is a picture of the surfaces of genus 0, 1, 2, and 3.) Geometer William Thurston (another Fields winner) made the daring conjecture that three-dimensional shapes, too, can be classified in a more complicated but equally structured way. Perelman has proved this conjecture, which has Poincaré as a straightforward corollary. That means, in turn, that we can think about proving general statements about three-dimensional geometry in a way that we can't hope to about beetles or graphs.
Perelman's work isn't important because of its applications. It won't help anyone build a bridge, aim a rocket, crack a code, or privatize Social Security. Mathematicians, no dummies, like to point out that, in some unspecified future, Perelman's theorem might pitch in to help with these problems in ways that aren't obvious now. But its real significance is like that of the fact that a times b is equal to b times a; it's a basic structural statement about how the world is organized. If you prefer order to chaos, that's something worth caring about.] ( slate.com )
Blog : The Compatibility | Brutally Hard Math Is Its Own Reward
http://www.purbalingga.co.cc
ReplyDelete[Purbalingga Blog]